Projects 2024
Mitigating Defect Modes in Josephson-Junction-Based Superconducting Qubits
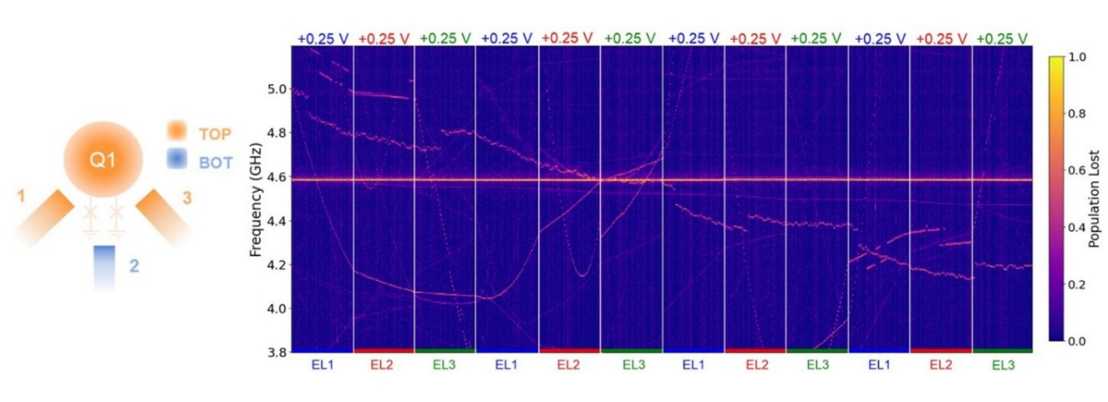
By: Zheng Liu, Emre Vardar, Giovanni Zhang
Host laboratory: Wallraff Group
In this project, the development and application of new on-chip technology have been addressed, with the intention to counteract two level system (TLS)-related defect modes in Josephson-junction-based superconducting qubits. Due to the destructive nature of TLSs on the coherence properties of the qubit, on-chip electrodes have been developed to produce electric fields inside and around the junctions that allow for local control of TLSs. As a result, we observed increased coherence in our qubits, coming from the systematic tuning of the defect modes. To counteract the collateral effects on the qubit’s coherence caused by the electrodes, 3D low-pass filters were designed. In this way, high-frequency noise was effectively suppressed, resulting in a higher coherence time of the qubit.
Automated Quantum Control Using Low-cost Actuators and Machine-supervised Beam Alignment
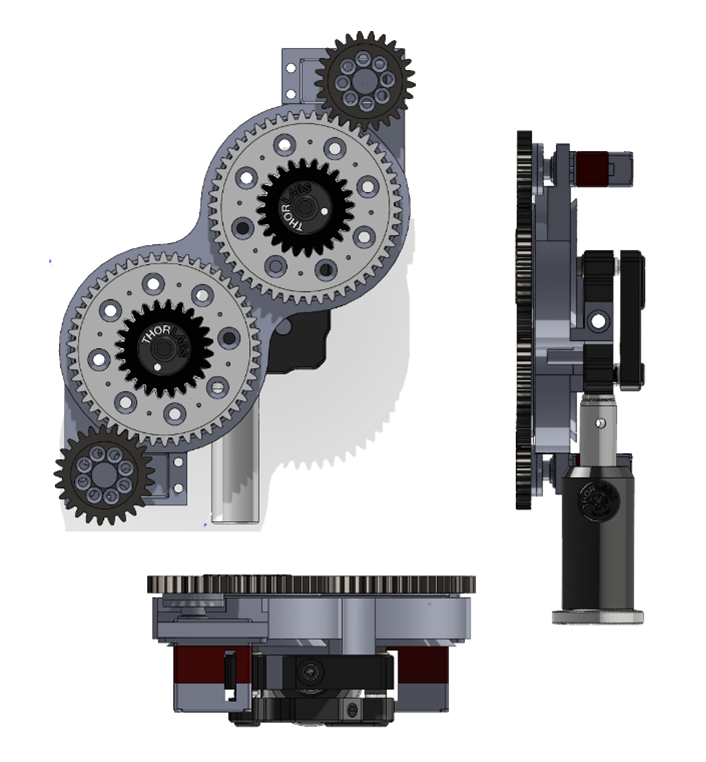
By: Debang Shi, Mattia Fiore, Matthias Kamm, Lennart Horn
Host laboratory: Xu Group
In recent years, Rydberg atoms have emerged as a promising qubit architecture for building quantum computers. To make Rydberg atom-based quantum computers capable of solving real world problems, they must be scaled to thousands of qubits. This project focuses on building an automated beam alignment system that is cost-effective and scalable. The system is implemented and tested on an optical conveyor belt setup that may be used in a Rydberg atom-based quantum computer for atom transport. Our system is split into a software and a hardware part. The hardware part consists of low-cost servo motors and custom 3d-printable couplers that connect the servo motors to the mirror mounts. The software part is designed to move the servo motors in an accurate and controlled manner. The main components of the software part are a closed loop feedback system and a neural network that can easily be retrained and finetuned in order to account for drifts or changes in the setup. Due to the combination of neural network and closed loop feedback, our system is capable of applying quick and accurate corrections even in highly non-linear systems.
Designing of a Compact Penning Trap with Integrated Optics
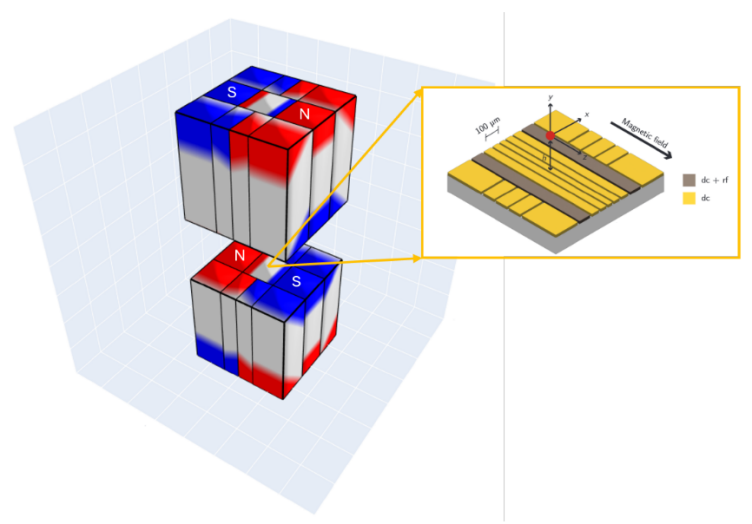
By: Zhuoyu Chen, Andrii Dumik, August Gude, Remi Truong
Host laboratory: Home Group
Trapped ions are a leading platform for quantum computing, offering state-of-the-art gate fidelities and record-long coherence times. Most trapped-ion quantum processors rely on Paul traps to confine and manipulate ions, but their inherently one-dimensional nature limits scalability. In contrast, Penning traps enable arbitrary ion arrangements in a two-dimensional plane, making them a promising candidate for large-scale quantum processors. In this Quantech Workshop, we have designed schemes for adiabatic ion transport in the magnetic field of a Penning trap. Using cleverly designed movement functions, we have simulated adiabatic transport over large distances and at high velocities. This is essential for ion-ion interactions when scaling up to a larger Penning trap array. Further, we made design improvements towards a compact Penning trap compatible with integrated optics. With permanent magnets in a Halbach array, we achieve the strong magnetic field required for ion confinement and eliminate the need for a bulky superconducting electromagnet. Simulations of the magnet assembly show that the field reaches 3T, with stability better than 0.1 ppm over a 1mm2 region. Combining the Penning trap with integrated optics for qubit addressing and readout enables scalable and parallelizable quantum operations.
Developing a Low-cost, Room-temperature Quantum Computer
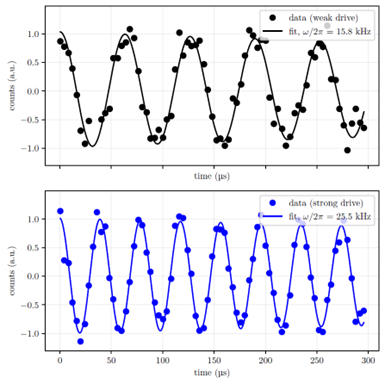
By: Robin Reding, Julian Santen, Alba Spahiu, Taiga Takahashi
Host company: external page Qzabre
The high cost and technical complexity of conventional quantum computing platforms often limit their accessibility for university and laboratory demonstrations. To overcome this challenge, we have developed a room-temperature quantum demonstrator based on the nitrogen vacancy (NV) center in diamond, a system that enables coherent spin control, robust readout, and straightforward operation under ambient conditions. Our work followed a two-stage approach, with both stages progressing concurrently. The first stage involved modifying a commercial confocal microscope (QZabre) to implement dynamical decoupling control of a 13C nuclear spin via the NV’s electronic spin, as well as direct radio-frequency (RF) driving of the 14N nuclear spin. We demonstrated coherent manipulation of these coupled spins, validating key requirements for universal quantum operations. In parallel, we constructed a custom table-top confocal setup using off-the-shelf optics and a home-designed XYZ stage for sub-micron sample positioning. This system successfully achieved optically detected magnetic resonance (ODMR) on an NV ensemble at room temperature, confirming the feasibility of low-cost quantum initialization, readout, and control. Our results demonstrate a viable approach for hands-on quantum experiments using accessible and cost-effective hardware, paving the way for broader adoption of quantum technologies in educational settings.
QuantumOverflow - Understanding Tsunami Risk with Quantum Algorithms
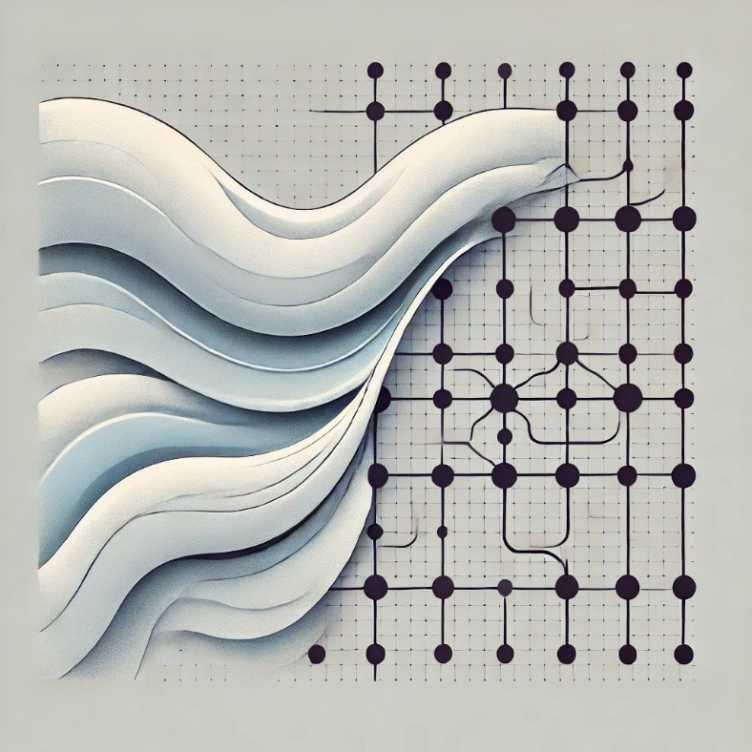
By: Petr Ivashkov, Franscesco Conoscenti, Zofia Binczyk, Till Appel
Host company: external page Moodys
Computational fluid dynamics lies at the heart of many issues in science and engineering, but solving the associated partial differential equations remains computationally demanding. With the rise of quantum computing, new approaches have emerged to address these challenges. In this work, we investigate the potential of quantum algorithms for solving the shallow water equations, which are, for example, used to model tsunami dynamics. By extending a linearization scheme previously developed for the Navier-Stokes equations, we create a mapping from the nonlinear shallow water equation to a linear system of equations, which, in principle, can be solved exponentially faster on a quantum device than on a classical computer. To validate our approach, we compare its results to an analytical solution and benchmark its dependence on key parameters. Additionally, we implement a quantum linear system solver based on quantum singular value transformation and study its performance in connection to our mapping. Our results demonstrate the potential of applying quantum algorithms to fluid dynamics problems and highlight necessary considerations for future developments.